Improved Liquid Scintillator For Radio-Flow Measurement
Kristie T. Schavey with R.A. Schavey and E. Rapkin
An informed guess suggests that 3,000-4,000 continuous-flow radioactivity monitors are currently in use. Almost all are interfaced to HPLC systems to measure biological material. They are all coincidence-type liquid scintillation counters with two opposed photomultipliers examining light produced by the interaction of radioactive decay events with a scintillator. Measurement cells are transparent small-volume (10-2000 µL) coils. Perhaps in one-third of installations, HPLC eluate flows through insoluble particulate scintillator packed within the coil. However, in the majority, a proprietary "liquid scintillator" solution containing various fluors - 2,5-diphenyloxazole (PPO) is most common - is continuously mixed with the eluate to produce the scintillations that are then measured.
Formulation of these scintillator solutions presents both obvious and subtle challenges. Simplified, the scintillation process involves radiationless energy transfer from the decay site within the liquid scintillator/mobile phase mixture to the dissolved scintillator via solvent molecules. Scintillators must fluoresce at wavelengths where photomultipliers are most responsive, i.e., in the ultra-violet around 400 nanometers. Solvents and additives must be transparent in that region.
For energy transfer, the best solvents are aromatic hydrocarbons, but HPLC eluates from biological separation normally contain considerable water, often with inorganic buffers. Therefore, detergents and emulsifiers are employed to achieve homogeneity; they must not seriously interfere with the scintillation process. Obvious mutual solvents such as alcohols are less useful since in necessary concentrations they inhibit the scintillation process.
With HPLC eluate flows of 1 mL/min or less, what is an appropriate scintillator flow? Several factors are important components of the answer - Fluidity, Performance, Cost, Disposal. They are each briefly considered:
- Fluidity - In Flow-Through detectors liquid scintillator and mobile phase come together at a tee fitting; to preserve eluate integrity, dynamic mixing is not employed. A liquid scintillator that forms stiff gels with the mobile phase at the interface, as many do, is useless even if vigorous agitation returns the mixture to fluidity. Too often, that is the case at low scintillator:mobile phase ratios. Sometimes gelling can be overcome by increasing the ratio, but too high a ratio creates Performance, Cost, and Disposal problems.
- Performance - A useful liquid scintillator provides efficient counting with low backgrounds at the working ratio. A ratio that is too high results in increased flow rates through the cell and shortened measurement time. The cell can only be made larger to accommodate increased flow at the expense of resolution, i.e., peak separation, and perhaps increased background.
- Cost - Scintillation solutions are expensive. The ability to work with low scintillator:mobile phase ratios may substantially reduce operating costs.
- Disposal - Unless the scintillator solution is biodegradable, hazardous waste disposal costs can approach and even exceed the cost of the original solution. Though biodegradability invites sewering, the presence of radioactivity may not permit it, thereby making a minimum volume scintillator more advantageous.
A new liquid scintillator has been developed that provides better answers to these problems than have been available in the past. "IN-FLOW 2:1", a proprietary mixture, provides fluid solutions, either clear or at worst hazy, with ratios as low as 1:1. It is biodegradable and, by virtue of the low ratios possible, operating cost is actually less than for less expensive solutions that must be used in greater quantity. Backgrounds are as low or lower than other scintillators that have been tested.
IN-Flow 2:1 is compared with three widely used commercial liquid scintillators with static samples (Table 1). For each of the two isotopes - 3H and 14C - the same amount of activity in 100 µL of water was pipetted into replicate vials containing 10 mL of the indicated scintillator:water ratio and counted in an automatic liquid scintillation counter:
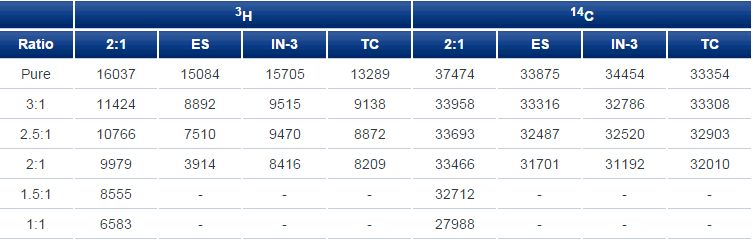
Table 1. Static Counting. ES (IN-FLOW ES), biodegradable scintillator solution for high levels of methanol and acetonitrile; IN-3 (IN-FLOW 3), for mildly buffered highly aqueous samples; TC (IN-FLOW TC), for high concentrations of ammonium phosphate and formate buffers.
For scintillators other than IN-FLOW 2:1, ratios of 1.5:1 and 1:1 led to milky-white mixtures that were not tested. From Table 1, it is evident that at all levels, and with both isotopes, IN-FLOW 2:1 was superior. With 14C being so much more energetic than 3H, 14C counting with all scintillators, and at all but the lowest ratios, is not much affected by increased water concentration. That is much less true for 3H where there are significant differences between scintillators including substantial concentration effects.
Turning to continuous-flow measurement where perhaps mixing, or rather lack of mixing, might be evident, we see (Table 2) strong confirmation of the static counting results for IN-FLOW 2:1. Fluid solutions were obtained and the fall-off in performance for 3H as the ratio is lowered almost perfectly parallels that observed under static conditions.
For scintillators other than IN-FLOW 2:1, ratios of 1.5:1 and 1:1 led to milky-white mixtures that were not tested. From Table 1, it is evident that at all levels, and with both isotopes, IN-FLOW 2:1 was superior. With 14C being so much more energetic than 3H, 14C counting with all scintillators, and at all but the lowest ratios, is not much affected by increased water concentration. That is much less true for 3H where there are significant differences between scintillators including substantial concentration effects.
Turning to continuous-flow measurement where perhaps mixing, or rather lack of mixing, might be evident, we see (Table 2) strong confirmation of the static counting results for IN-FLOW 2:1. Fluid solutions were obtained and the fall-off in performance for 3H as the ratio is lowered almost perfectly parallels that observed under static conditions.
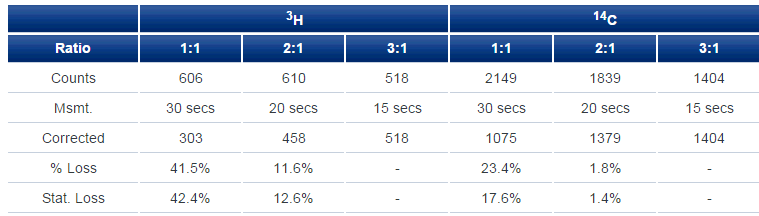
Table 2. Flow counting with IN-FLOW 2:1. 1 mL/min of water together with IN-FLOW 2:1 in the indicated ratio was pumped through a 1000 µL cell. Six replicate 7 µL injections of radioactive solution were made. Averaged raw counting results are presented (counts per peak) as are values corrected for differences in counting time referenced to 3:1 ratio. Loss in counting efficiency, also referenced to 3:1 ratio, is compared for flow and static counting.
In addition to pure water, methanol/water and acetonitrile/water are widely used mobile phases. Comparative static counting of 3H with the four scintillators confirms the superiority of IN-FLOW 2:1 (Table 3).
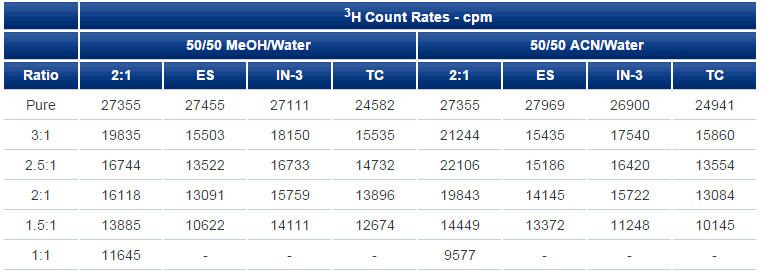
Table 3. Static counting comparison of IN-FLOW 2:1 with other scintillators. The same amount of 3H activity was pipetted into each counting vial.
While subjective, with IN-FLOW 2:1, 2:1 is probably the ideal ratio considering performance, cost, and the potentially longer counting time in flow, leading to more total counts and better statistics.
Conclusion
IN-FLOW 2:1 has provided encouraging results that merit further study. When an HPLC mobile phase is all water or 50/50 water-methanol or water-acetonitrile, it is clearly superior to a spectrum of commercial scintillator solutions. Beta testing under actual use conditions with buffers and other common components found in HPLC has confirmed the superiority of IN-FLOW 2:1 to earlier scintillator formulations. IN-FLOW 2:1 offers not only the prospect of substantial economy, but also of greater sensitivity as combined flow rates are lessened and counting times thereby increased.